Our work on active hydrodynamics aims at identifying generic aspects of the large scale behavior of active systems and characterizing their materials properties. Many of the macroscopic properties of active systems are universal in the sense that systems operating at widely differing length scales, with significant differences in their detailed dynamics at the microscopic level of the self-propelled units, display broadly similar properties. Visually similar flocking phenomena are seen in fish shoals and collections of keratocytes. Contractile stresses are seen on a sub-cellular scale in the cytoskeleton, as well as on a scale of many cells in swimming algae.

In our recent work we have focused on “dry” active systems, with no momentum conservation as may be realized by active particles moving on a frictional substrate or through a porous medium, and on understanding the interplay of orientational order and flow in controlling the large scale behavior of the system. Active particles are generally elongated and form orientationally ordered states. The nature of the ordered state depends on both the symmetry of the individual particles and the symmetry of the aligning interactions, as sketched in the figure. Physical realizations of polar active particles (with a head and a tail, often molded as self-propelled (SP) particles) include bacteria, asymmetric vibrated granular rods, and polarized migrating cells. Apolar (head-tail symmetric) active particles, often referred to as “shakers”, have also been considered in the literature. Realizations are symmetric vibrated rods and perhaps melanocytes, the cell that distribute pigments in our skins.
The nature of the interaction is of course crucial in controlling the properties of the ordered state. Apolar active particles generally experience apolar interactions and the resulting ordered state has the symmetry of equilibrium nematic liquid crystals. SP and polar particles with polar interactions, i.e., ones that tend to align particles head to head and tail to tail, order in polar states with ferromagnetic symmetry, where the ordered state is also a moving state, as in the Vicsek model.
Our group has recently identified and studied a third class of active fluids, consisting of SP particles with apolar interactions (such as steric repulsion or hydrodynamic interactions among swimmers in a suspension), where the emergent macrostates are nematic in symmetry. The properties of this self-propelled nematic fluids are distinct from those of active nematics composed of apolar particles. t has recently become apparent that models of polar particles with apolar interactions may be relevant to a number of physical systems, including gliding myxobacteria, suspensions of self-catalythic Janus colloids and motile epithelial cell sheets such as those studied in wound healing assays.
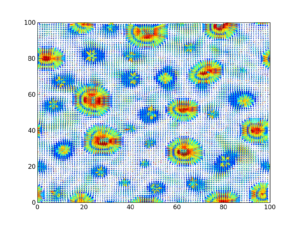
We are currently exploring these models in several new directions. One area of investigation is the role of density dependent mobility of active particles, as may arise from steric effects and local crowding or from biochemical signaling such as quorum sensing in bacterial colonies, in controlling emergent behavior. A second one is the effect of cell division and death that eliminates particle number conservation and dramatically modifies the dynamics. Finally, a third area of focus is on making contact between theoretical models and physical systems.